Quantum Computing
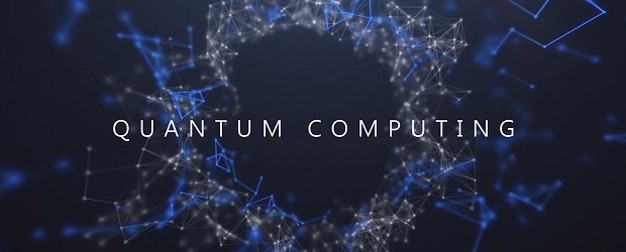
Scientists won't be able to use standard computers to perform tasks like harness the power of molecules during photosynthesis. They must make use of quantum computers, which can calculate the conditional probability of events and measure and observe quantum systems at the molecular level. In essence, quantum computers have the ability to do billions of years' worth of computation in a single weekend, solving some of the most challenging issues in existence.
Quantum computing is a rapidly-emerging technology that harnesses the laws of quantum mechanics to solve problems too complex for classical computers.
WHAT IS QUANTUM COMPUTING?
The practice of using quantum physics to tackle issues that are too difficult or big for conventional computers is known as quantum computing. Qubits are essential for running and resolving multidimensional quantum algorithms on quantum computers. A machine that works on the laws of quantum mechanics, behavior of particles at the sub atomic level.
Using the principles of quantum theory, quantum computing runs quantum models and solves mathematical problems. It is used to simulate various quantum systems, such as photosynthesis, superconductivity, and intricate molecular structures.
Supercomputers aren't all that super for some issues
Supercomputers are used by scientists and engineers when they are faced with challenging tasks. These are enormous classical computers that frequently have thousands of cores for both the CPU and GPU. Nonetheless, some types of issues are difficult for even supercomputers to solve.
When a supercomputer struggles, it's often because the large classical machine was given a challenging problem to answer. Complexity is commonly to blame for the failure of traditional computers.
Many variables that interact in intricate ways are considered complex problems. Because there are so many different electrons interacting with one another, modelling the behavior of individual atoms in a molecule is a challenging task. It is difficult to determine the best paths for a few hundred tankers in a vast transportation network.
You must first comprehend qubits, superposition, entanglement, and quantum interference in order to comprehend quantum computing and how it operates.
Quantum computing uses the superposition and entanglement aspects of quantum physics to carry out computation. Binary encoding is used by conventional transistors to store data that is electrically represented as "on" or "off" states. Unprecedented levels of parallelism and computing efficiency are made possible by the ability of quantum bits, or "qubits," to simultaneously operate in several states.
Quantum systems available today can only solve practical issues with tens or hundreds of entangled qubits. Commercial quantum systems need to scale to over a million qubits and overcome difficult obstacles like qubit fragility and software programmability in order to become quantum practical.
WHY DO WE WANT QUANTUM COMPUTERS?
Engineers and scientists believe that quantum computers will be able to solve some issues that are practically impossible for traditional, classical computers. It is also anticipated that quantum computers would undermine present cryptography techniques and open up fresh avenues for truly secret communication.
We will be able to study, simulate, and control other quantum systems with the aid of quantum computers. This capability will enhance our comprehension of physics and have an impact on the designs of items such as computer chips, communication devices, energy technologies, scientific instruments, sensors, clocks, and materials that are engineered at scales where quantum mechanics is relevant.
HOW DOES A QUANTUM COMPUTER WORK?
There are some similarities between classical and quantum computers. For instance, chips, circuits, and logic gates are typically present in both types of computers. They use a binary code of ones and zeros to represent information, and their operations are guided by algorithms (basically sequential instructions).These ones and zeros are encoded using tangible items in both types of computers. These devices are used in traditional computers to encode bits (binary digits) in two states, such as whether a magnet is pointing up or down or if a current is flowing.
Quantum computers use quantum bits, or qubits, which operate fundamentally differently from traditional computers. A qubit can concurrently represent one and zero, whereas classical bits can only represent one or zero, at least until their state is measured. Moreover, several qubits' states may be entangled, which means they are connected quantum mechanically. Quantum computers have abilities not available to classical computers thanks to superposition and entanglement. Qubits can be created by manipulating atoms, electrically charged atoms known as ions, or electrons. They can also be created by Nano engineering 'fake' atoms, like circuits of superconducting qubits, using lithography, a printing technique.
WHAT ARE QUBITS?
The fundamental piece of information in quantum computing are quantum bits, or qubits. similar to a standard binary bit used in old-school computers. Qubits can be in numerous states at once thanks to superposition. Only 0 or 1 can be represented by a binary bit. Any portion of 0 or 1 in a superposition of the two states, as well as 0 or 1, are all possible for a qubit.
HOW ARE QUBITS CREATED?
The answer depends on how quantum systems are built, as some need very low temperatures to operate effectively. While binary bits are frequently generated from silicon-based devices, qubits can be made from trapped ions, photons, artificial or actual atoms, or quasi particles.
In a digital computer, information is stored and processed using bits, which can either be 0 or 1. Physically, anything that can be configured in two different ways—one represented by "0" and the other by "1"—is considered to be a bit. It could be a system having two different and distinguishable outcomes, such as a on or off light bulb, a heads or tails coin, or any other system. The lack or presence of an electrical signal, which encodes "0" or "1," serves as the bit representation in contemporary computing and communications.
Every bit made from a quantum system, such as an electron or photon, is referred to as a quantum bit. A quantum bit must have two different states, one representing "0," and the other representing "1," much like classical bits. A quantum bit can be entangled with other quantum bits and exist in superposition states, in addition to being sensitive to measurements that are incompatible with it. Qubits are fundamentally distinct from and significantly more powerful than conventional bits due to their capacity to harness the powers of superposition, interference, and entanglement. We require quantum objects that function as qubits in order to construct quantum computers and other quantum information technologies. Several physical systems can now be harnessed and controlled by science to function as qubits. This enables us to balance the benefits of each type of qubit with the requirements of various quantum technologies.
WHAT IS SUPERPOSITION?
Some people mention Schrödinger's cat when explaining superposition, while others mention the brief moments while a coin is in the air during a coin flip. Quantum superposition, to put it simply, is a mode in which quantum particles combine all conceivable states. The quantum computer measures and tracks each particle while it is still moving and fluctuating.
Instead of concentrating on the ability to have two things happen at once, superposition has the advantage of allowing for numerous perspectives and a variety of inquiries. In other words, unlike regular computers, quantum computers are capable of doing a huge number of parallel computations simultaneously.
We can only really simplify things so much before we start reciting equations. The overall conclusion is that superposition is what enables a quantum computer to "try all pathways at once.“
Quantum objects can exist in multiple states or locations at once thanks to superposition. This implies that an object can exist in two states simultaneously and still be a single object. As a result, we can investigate significantly richer collections of states.
WHAT IS ENTANGLEMENT?
Entanglement is the term used to describe the ability of quantum particles to coordinate measurements with one another. Measurements from one qubit during entanglement can be utilized to draw conclusions about other units. Quantum computers can calculate and solve larger stores of data and information because to entanglement.
Qubit entanglement and superposition probabilities are used by quantum computers to carry out operations. We may modify these processes to make specific probabilities higher or lower, which gets us the right and wrong answers we're looking for. Quantum computers have a lot of flexibility in the directions they can go.
There are three essential components of a quantum computer:
A component containing qubits; A component responsible for signaling the qubits; A conventional computer that can execute programmes and issue commands
Quantum particles can be manipulated in a variety of ways, as was previously indicated. To improve coherence and reduce interface in some quantum computers, the portion that houses the qubits is kept at a very low temperature. Some quantum computers keep the portion that houses the qubits in a vacuum chamber, which lessens vibrations and aids in maintaining the balance of the qubits. Microwaves, lasers, and voltage can all be used by the component of the quantum computer that sends signals to the qubits to send those signals.
QUANTUM COMPUTERS VS CLASSICAL COMPUTERS
Quantum computers employ qubits to process information, as opposed to traditional computers, which use transistors to represent the binary numbers 0 or 1. Qubits can simultaneously represent 0 and 1, according to the superposition principle.
Quantum computers' processing capacity increases exponentially as the number of linked qubits increases. In contrast to classical computing, this occurs. A traditional computer's power grows linearly as the number of transistors increases.
Applications: Complex activities including optimization issues, data analysis and processing, and simulations are better suited for quantum computers. Our daily processing requirements are best served by traditional computers.
constructing blocks The fundamental components of quantum computers are superconducting quantum interface devices (SQUID), often known as quantum transistors. CMOS transistors are used in traditional computers.
Data processing: The Quantum Processing Unit (QPU), which is made up of connected qubits, is where data processing in quantum computing takes place. The Central Processing Unit (CPU), which is made up of the Arithmetic and Logic Unit (ALU), processor registers, and a control unit, is where data processing takes place in traditional computing.
Speed: Compared to conventional computers, quantum computers are hundreds of millions of times faster at solving some problems.
QUANTUM SUPREMACY & THE ROLE OF QUANTUM PHYSICS
Quantum supremacy is a goal that several groups engaged in quantum computing are working towards. A quantum gadget that can solve a task that no classical computer can in a workable period of time is said to be capable of quantum supremacy. Despite the astounding achievements of modern quantum computers, we have yet to demonstrate quantum superiority for practical, everyday tasks.
QUANTUM INTERFERENCE
Superposition produces a consequence known as quantum interference. It enables us to bias a qubit's measurement towards a particular state or group of states. Keep in mind that because to superposition, a qubit can be either zero or one, or both, simultaneously. Depending on how they are organized, qubits have a chance of exploding to zero or one. Quantum interference determines this probability. In essence, quantum interference enables us to modify a qubit's state to modify the likelihood of the desired result.
QUANTUM ENTANGLEMENT
Entanglement is one of the quantum features used in quantum computing. Two or more quantum particles can entangle due to quantum entanglement. These particles merge into a single system when they get entangled. This indicates that the entanglement's quantum particles are all described as a single entity. Because it increases the number of qubits in a system, quantum entanglement increases the computing capacity of qubits. Every time we perform an operation on one particle, the other entangled particles are also affected.
QUANTUM DECOHERENCE
The feature of quantum physics that prevents quantum computing from progressing is quantum decoherence. The superposition state may collapse when we attempt to view or measure quantum particles. We refer to this as decoherence. In quantum computational systems, quantum decoherence causes errors. That makes it challenging to maintain superposition's for long enough to carry out calculations that are genuinely helpful. The term "coherence length" describes how long a qubit may maintain its quantum characteristics. We need to employ quantum error correction to extend this length and create fault-tolerant quantum computers (QEC). By resolving decoherence errors, QEC can increase coherence length.
HOW DO QUANTUM COMPUTERS WORK?
Compared to conventional computers, quantum computers operate in a fundamentally different manner while processing data. Although conventional computers use binary bits to communicate information, quantum computers use qubits. The core of quantum's potential for exponentially more powerful computation is the qubit's capacity to remain in superposition. A variety of algorithms are used by quantum computers to perform measurements and observations. After the user enters these methods, the computer constructs a multidimensional space where patterns and specific data points are stored. For instance, the quantum computer would measure the combinations of folds; this combination is the solution to the problem if a user wanted to solve a protein folding problem to find the least amount of energy to consume. A quantum computer's physical appearance can differ.
A real quantum computer is physically composed mostly of three components. The first component consists of a conventional computer and supporting infrastructure that executes programmes and communicates with the qubits. A technique for sending signals from the computer to the qubits is the second component. And finally, the qubits need to be stored somewhere. Some demands or conditions must be met for this qubit storage unit in order for the qubits to be stable. This can include the necessity for temperatures to be close to absolute zero or the interior of a vacuum chamber.
It turns out that qubits require more upkeep than even the most prone to meltdown rock star. Simple operations or changes in variables might cause error-prone qubits to lose their quantum state or slip into decoherence. A quantum computer may crash as a result of measuring qubits or performing operations. Or, put another way, employing it. Qubits will also begin to lose coherence in the presence of even slight vibrations and temperature changes. Because of this, superconducting circuit-based quantum computers, which are the most common type and are favored by Google and IBM, must be kept isolated and at extremely low temperatures (a cool -460 degrees Fahrenheit).
The difficulty is dual. The fidelity of each physical qubit needs to be improved first. Better engineering, establishing the ideal circuit layout, and discovering the ideal component combinations could all lead to that. After that, we must organize them to create logical qubits. One fault-tolerant qubit is thought to require anywhere from hundreds to thousands to tens of thousands of physical qubits. Without a doubt, none of our current technologies could scale up to those levels. To manage the rise in qubit quality and number, researchers would also need to create ever-more sophisticated systems.
WHAT CAN QUANTUM COMPUTING SOLVE?
The issues and difficulties that can be solved by quantum computing are as varied as the states that qubits can exist in. Quantum computing has a variety of applications, including search, probability, molecular simulation, and optimization. By using QCs to execute quantum-inspired algorithms, problem solving can be enhanced by quantum computing. Due to their reliance on elements like cost, quality, and production time, the scientific and industrial areas can both benefit from these optimizations. There will be advancements made in the management of energy storage, package delivery, air traffic control, and other areas thanks to quantum computing.
The largest molecule to date to be modelled on a quantum computer was beryllium hydride, which was done in 2017 by IBM researchers. In 2019, IonQ researchers took another significant breakthrough by modelling a water molecule using quantum computing. Typically, these are still minor issues that may be verified with conventional simulation techniques. But, things are advancing that will be challenging to verify without actually constructing a sizable particle physics experiment, which might be very expensive.
There is also optimism that massive quantum computers will speed up AI and vice versa, however experts are divided on this issue. We can't simply adapt algorithms from conventional computers to quantum computers since the laws are completely different, at the most fundamental level. This is why there is dispute. Some people think that by enhancing carbon capture, quantum computers can help fight climate change. If successful, quantum modelling of bigger molecules could contribute to the development of a catalyst for directly 'scrubbing' carbon dioxide from the environment.
We are still in the NISQ era, which stands for Noisy, Intermediate-Scale Quantum. In a word, quantum "noise" makes it extremely challenging to stabilise such computers. NISQ computers are now utilised largely for research and teaching because they can't be relied upon to make judgements with significant commercial implications.
WHY QUANTUM COMPUTING IS IMPORTANT & THE FUTURE ROADMAP
Some of our current systems might be destroyed by quantum computers. From email to online retail transactions, the RSA cryptosystem offers the security framework for a variety of privacy and communication protocols. In order to maintain current standards, it is assumed that no one has the processing power to evaluate every method for decrypting data once it has been encrypted. Nevertheless, a mature quantum computer could test every method in a couple of hours.
It is important to note that quantum computers have not yet reached this stage of development and won't for some time, but if and when a sizable, reliable device is created, its unmatched capacity for factoring enormous numbers would essentially destroy the RSA cryptosystem. Fortunately, the technology is still in the future, and researchers are working on it.
Even though quantum computing is still in its picky, disagreeable stage, business interests have jumped in. During the 2020 Consumer Electronics Show, IBM declared that its 'Q Network' had grown to include more than 100 businesses and organizations. Now, partners include Mercedes-Benz owner Daimler AG, Anthem Health, and Delta Air Lines.
Some of those collaborations are dependent on quantum computing's potential for molecular simulation. For example, Daimler is expecting that technology will eventually lead to a better technique to make batteries for electric vehicles. However far off it may still be, agreements between quantum computing startups and top pharmaceutical firms like those formed by ProteinQure and AstraZeneca and 1QBit indicate to the potential of quantum molecular modelling for drug discovery.
To calculate the chemical properties of a novel substance, researchers would require millions of qubits. A quantum computer already understands quantum mechanics, so we can essentially programme in how another quantum system would behave and use it to echo the other one.
Another unanswered question is which quantum computing technique will prevail. Even while superconducting has so far produced the most results, scientists are looking into alternate techniques like trapped ions, quantum annealing, and so-called topological qubits. Finding the optimal strategy for various applications is more important, in Donohue's opinion, than deciding which technology is superior. For instance, the magnetic field technology that supports neuroimaging readily fits with superconducting chips.
Yet, there are difficulties with quantum computing that go beyond hardware. The "magic" of quantum computing is not in speed but in algorithmic developments.
The widespread lack of knowledge is yet another barrier to quantum computing. Simply said, there aren't enough.
QUANTUM COMPUTING USE CASES
Search: Unstructured data searches might have their solution accelerated by quantum algorithms.
Quantum simulation: Because quantum phenomena are a part of quantum computation, quantum computers are able to simulate other quantum systems. Hence, more intricate quantum systems, including photosynthesis and superconductivity, might be simulated.
Quantum computing can assist us in solving our optimization-related issues. In order to identify better ways to control complicated systems, such package delivery and traffic flows, we can use quantum optimization techniques.
Cryptography: Quantum algorithms may be able to decrypt keys used in classical cryptography, which are now too complicated for conventional computers to decrypt.
Healthcare: The application of quantum computing may help to advance areas such as cost, diagnostic support, imaging, and precision medicine.
Finance: By accelerating crucial financial calculations, quantum algorithms could help us make more accurate estimates.
Chemical and biological engineering: Quantum mechanics is used in chemical and biological engineering to move and interact. One of Richard Feynman's primary driving forces behind the development of a quantum computer was the need to emulate quantum mechanics. Engineers might use quantum simulations to forecast new molecules' properties, which would aid in the development of pharmaceuticals and new materials.
Artificial intelligence: We could use quantum computing to process enormous volumes of data to improve the quality of our judgements and predictions in the field of AI. A developing subject called quantum machine learning examines how quantum algorithms can hasten AI projects.
QUANTUM COMPUTING & PROTECTING INNOVATIONS THROUGH INTELLECTUAL PROPERTY
Quantum computing was ranked among the top 10 fastest-growing technologies last year according to an analysis of U.S. patent filings.
A $1 trillion industry?
It's hard to imagine, but quantum computing will eventually solve issues that are thought to be too challenging or impossible for existing systems. Over the past few years, patent applications in this field have increased dramatically to keep up with the escalating commercial opportunities and private finance. Quantum computing will rank among the top 10 fastest-growing technologies in 2020, according to an analysis of U.S. patent filings.
IBM, Google, Northrop Grumman, D-Wave Systems, and Microsoft are among of the top candidates. Industry players should be aware of the practical limitations of trade secret, copyright, and patent protections as they seek to safeguard their ideas.
PATENT PROTECTIONS
There are already more than 200 U.S. patents on quantum computing. Their research interests include machine learning, supply chain optimization, financial asset portfolio optimization, molecular simulations for pharmaceutical development, and quantum hardware error correction.
Patents have defensive utility and can be bought, traded, or licenced. Patent wars are frequently started by land grabs for patents in disruptive technology. Quantum computing standards are already being developed by standard-setting bodies, and individuals who hold patents that are crucial to the standard but are unwilling to licence their technology could demand royalties from those who use it.Even individuals who aren't engaged in aggressive litigation may benefit from using patents as a negotiating tactic or to prevent rivals from acquiring interfering intellectual property rights, preserving their freedom to operate.
PROBLEMS WITH PATENTABILITY MAKING ENFORCEMENT DIFFICULT
Nonetheless, some patentability difficulties can make prosecution or enforcement more difficult. Improvements to hardware usually accompany quantum computing advancements; in these situations, prospective rejections frequently result from obviousness under 35 U.S.C. 103 or a lack of originality under 102. A few developments in quantum computing might possibly be excluded from the scope of Section 101.
Natural events and abstract concepts, such as mathematical formulas, are examples of subject matter that is not eligible for patent protection. Some assertions may be problematic because quantum computing relies on algorithms that take advantage of the superposition and entanglement quantum mechanical phenomena. A 101 denial was previously upheld by the U.S. Patent Trial and Appeal Board on the basis that quantum entanglement is a natural occurrence.
Courts decide that technological advancements like computers can signify such integration when an abstract idea or natural phenomenon is incorporated into a practical application. Yet, even if they are focused on a useful use, quantum computing claims shouldn't be so wide as to cover what the courts have referred to as software per se.
Examiners commonly reject claims under 101 as embracing non-statutory subject matter because software per se does not fit into any of the categories of patent eligible subject matter listed in 101. When a claim's broadest possible interpretation encompasses software per se. Claims to quantum software would benefit from clearly encapsulating tangible, non-transitory matter, with hardware elements incorporated where possible, to decrease such danger of rejection.
COPYRIGHT PROTECTION
As an alternative, quantum software is covered by copyright laws. Software copyrights, which can be secured by registering, provide the creator exclusive rights for as long as they are alive plus an additional 70 years. When an employee develops the work while employed, these rights are assignable and typically belong to the employer. The speed of copyright registration, which can be sped up to just a few days, complements the rights acquired at the end of a protracted patent prosecution cycle in a sector that experiences rapid change.
DIFFICULTIES DETECTING INFRINGEMENT
Enforcing quantum computing patents may be challenging due to difficulty in identifying infringement, in addition to issues with patentability. As a large portion of current quantum computing is cloud-based, demonstrating infringement may involve reverse engineering, but quantum hardware may be unreachable.
Reverse engineering may also be made more difficult by the fact that quantum computation takes place in a superposition of states, which is destroyed when viewed, and that logic operations are only intended to measure results. Limitations on patent protection may make secrecy a preferable option.
TRADE SECRET PROTECTION
Another technique to preserve ideas is through trade secret protection for technologies that are difficult to reverse-engineer. This defense starts up automatically and doesn't expire; it lasts until the secret technology is found on its own, deciphered, or made public by the owner. The protection is automatic, but businesses can take legal action to make sure the secret is kept a secret, including prosecuting those who do. Many businesses view trade secret protection as having a certain advantage over patent protection because to the greater subject matter and potentially endless years of protection.
While patents typically last for 20 years, quantum computing is still in its early stages, and it may be more than ten years before quantum computers are widely available commercially. In cases where inventions might not become commercially viable during the term of a patent, trade secret protection may be more advantageous. On the other hand, technological advancement might quicken in the future to the point where a patent would be out of date by the time it was granted. The protection provided by copyright law can also occasionally be limited by courts due to a variety of doctrines, so trade secrets may be a better way to safeguard an innovation.
While various businesses decide on the approaches they believe will best safeguard their innovations in light of anticipated go-to-market plans, a balanced combination of information security regulations along with a targeted intellectual property strategy will help guarantee their innovations a place in the quantum computing future.
Please feel free to reach out to one of our subject matter experts at info@effectualservices.com
to explore, how we can help you & make the world a better place to live in !!!
Effectual’s QUANTUM COMPUTING RESEARCH FRAMEWORK is a deep dive into this ecosystem and shall help you understand the intricacies of this nascent innovative domain with insights backed with credible data sources. Some ways we can help include, but not limited to - Performing any previous art or freedom to operate searches to help you better grasp the environment surrounding your invention or business endeavors. If certain methods of IP protection are more appropriate for your technological or business goals, we can help you strategize effectively to plan for future & in making continuous innovation a part of your working model.