Flexible Batteries
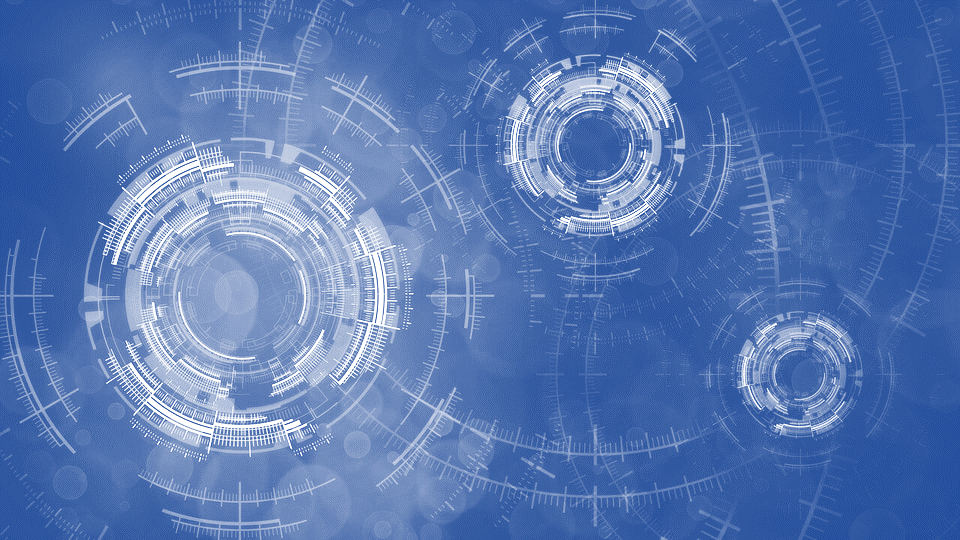
Prelude
A sizable, rectangular residue occupies around 40% of the interior of each consumer electronic device, including the iPhone, Samsung Galaxy, or any other. This is the battery.
Even if a strong and long-lasting battery is undoubtedly a crucial component of mobile and wearable technology, the current generation of lithium-ion batteries is what prevents our smartphones and fitness trackers from being much, much smaller. Flexible batteries, however, are also vying for attention at a time when flexible electronics are dominating the semiconductor debate.
Flexible sensors and devices are increasingly in demand. It's not surprising that significant funding is starting to flow into pertinent research given the numerous obvious applications for the flexible battery (things like wearable, mobile, and other personal electronic devices), as well as some exciting potential applications (defense and health care are also exciting possibilities).
Even more thrilling than what has already arrived is what is still to come. According to IDTechEx, the market and technological landscape are still quite complicated, with both of these factors "in a constant state of flux."
Nevertheless, the semiconductor industry will undoubtedly endeavor to power it with the newest batteries as flexible and stretchable technology points the way.
The research sector is currently valued $7 million but is projected to be worth $400 million by 2025.
Bring up ROI.
Introduction
A large need for flexible power sources has emerged in tandem with the quick development of flexible and wearable electronic gadgets, which has in turn prompted significant research and development into flexible batteries. High electrochemical performance and superior mechanical deformabilities are both essential for the optimal flexible battery. Therefore, key factors that should be carefully studied include battery core components, chemical systems, device layouts, and practical applications.
In recent years, flexible and high energy density energy storage devices have grown quickly in response to the rising need for wearable and flexible electronic items.
The most promising battery technology for wearable electronics is the flexible battery, which has a high energy density and reliable electrochemical performance in a variety of energy storage devices.
But lithium-ion batteries are now the most adaptable type of battery. Due to the fact that it only has a whole anode, separator, and cathode stack, its total performance is not as good as that of a rigid battery.
New electrolyte materials with excellent ionic conductivity, chemical inertness, mechanical stability, and flexibility are being developed by the top 5 battery electrolyte firms. This new type of flexible battery has great flexibility and mechanical resistance.
Advantages of flexible battery
Flexible batteries should provide the following advantages over traditional batteries:
Withstand stretching, bending, and folding
Wearable, flame-resistant, and waterproof
Self-healing abilities
Impact damage mitigation
Because of this, every part of a battery, such as the electrodes, electrolytes, current collectors, etc., need to be changed from conventionally rigid or brittle materials into a ductile matrix. A flexible battery can often only undergo one type of deformation at once, either bending deformation or twisting deformation.
The length of the connecting component is adequate for batteries with cylindrical cells, enabling the battery to undergo complex bending and twisting. Flexibility is assessed using a higher standard known as winding. Batteries with cylindrical units offer greater versatility because of their rounded shape.
Composition of the flexible battery
To avoid battery failure, some battery cells should also take into account mechanical stability, hydrophobicity, and flame retardancy. Additionally, each component should be securely attached in the order that it was intended to be. It primarily consists of the flexible anode, electrolyte, cathode material, and interlayer design.
Flexible anode - Pure lithium metal breaks when bent, folded, or stretched repeatedly because it is soft (0.6 on the Mohs scale), lacks plasticity (approximately 50–70% ductility), and cannot be repaired. The practical use of flexible lithium metal batteries is hampered by their low mechanical flexibility, high reactivity, and dendrite formation at lithium metal fissures.
Non-fluid electrolytes - New architectures have been created in response to the current demand for lithium metal batteries that are highly safe, light, durable, and ecologically friendly. The design and assembly of wearable electronic devices are challenging due to the danger of leakage from batteries using traditional liquid electrolytes and the susceptibility of electrode materials to corrosion.
Solid electrolytes (SSEs), gel polymer electrolytes (GPEs), and quasi-solid electrolytes (QSEs) are non-fluid types of electrolytes and can all be referred to as non-fluid electrolytes when compared to pure liquid electrolytes.
Lithium dendrites are more consistently prevented from growing by non-liquid electrolytes, enhancing battery safety and deformation. A solid polymer electrolyte, an inorganic-polymer composite solid electrolyte, and an inorganic solid electrolyte are included in the solid electrolyte in this case.
Electrochemical properties of flexible battery
Batteries with prismatic cells are flat and have slow capacity deterioration. Because of its innovative construction, this flexible battery is very adaptable to different deformations. A matching flexible battery can go through tens of thousands of dynamic cycles throughout operation because the majority of flexible electronic devices experience dynamic deformation while in use.
Since electrochemical performance and endurance are fundamental requirements, flexible batteries face significant difficulties in real-world scenarios. The innovative architecture prevents a large decline in battery capacity.
Flexible batteries with cylindrical units can tolerate more severe and complicated deformations because of the ring's intrinsic qualities. This flexible battery nevertheless performs about the same electrochemically. Once more, there is no discernible decrease in capacity. With various cycles, the corresponding charging and discharging platforms remain essentially unaltered, demonstrating the high mechanical stability and resistance of this flexible battery.
The stability of the battery under dynamic composite deformation is also very essential because flexible equipment typically doesn't deform at all when in use. The metal current collector may also always be deformed elastically because to its innovative construction, giving the flexible battery remarkable mechanical and electrochemical stability.
Current existing problems
In comparison to lithium-ion, lead-acid, nickel-metal hydride, zinc-manganese, and other energy storage technologies, lithium metal batteries have a theoretically higher energy density.
Although research has become more popular recently, the top 10 manufacturers of lithium ion battery anode materials are still in the early stages of developing flexible batteries.
Real energy density and flexibility indicators are the major indicators for measuring flexible lithium metal batteries while taking into account real-world applications. The following are essential among them:
Creation of anodes with top-notch electrochemical capabilities.
Creation of novel electrolyte materials with excellent ionic conductivity.
Increased elemental and flexible use of high-capacity cathode materials.
Design for a reasonable battery configuration.
To meet wear requirements, incorporate safety features including impact resistance, self-healing, waterproofing, and fire resistance.
Recent advances & road ahead
While there has been much recent research on the development of flexible or wearable electronic devices that can be integrated into clothes, glasses, watches and even skin, a bottleneck in this technology appears to be the power source. Thus, one of the most significant issues impeding the success of these newly developed portable gadgets is the development of flexible electrochemical energy-storage devices, which can convert chemical energy and electricity, reversibly.
Currently, flexible batteries, especially lithium-ion batteries (LIBs), are the best options for this use. When deformed in a variety of ways, such as stretching and bending, flexible batteries maintain their electrochemical performance.
Up until this point, bendable LIBs have received the most reports on flexible batteries. The limitations of bendable batteries involve electrodes, electrolyte, and other parts that are often produced from stiff materials, much like the fundamental problems for flexible electronics. Active materials for LIBs have typically been deposited on a flexible substrate (such as paper, cloth, plastic, carbon nanotube (CNT) and graphene films, etc.) to achieve a specific bendability in order to generate a bendable electrode.
These non-conductive substrates (paper, cloth, and plastic) have higher elastic properties, which is one of their main advantages. Non-conductive substrates, on the other hand, typically exhibit poor electrical conductivity and more electrolyte side reactions. Flexible electrodes made of CNT or graphene have clear advantages over non-conductive substrates in terms of electronic and ionic conductivity, although they are less stretchable.
Another barrier to the use of flexible LIBs is the electrolyte. A appropriate gel polymer or solid-state ceramic electrolytes with acceptable flexibility must be utilized to prevent the potential leakage of liquid electrolytes. For instance, gel polymer electrolytes may be the perfect component for flexible LIBs since they offer great interfacial contact in addition to sufficient mechanical flexibility.
The stretchable LIB is another variety of flexible LIB. Stretchable batteries are particularly challenging to develop since they must allow for both bending and stretching. Two methods are typically used to give electrodes stretchability. One is based on the materials themselves, including different active polymer polymers with sufficient stretchability and reversible lithium storage capacity. Unfortunately, implementing this method is exceedingly tough. The other is to employ creative structural design to stretch brittle inorganic materials, for as by utilizing electrodes with wavelike, fibrous, or island-bridge structures.
These recently created structures have the potential to break through the elastic limit of brittle materials and pave the way for stretchy technology. These newly created stretchable electrodes have an elastic limit that can go up to 1000%. CNTs and graphene have a great deal of potential to be employed in the construction of stretchable electrodes due to their superior mechanical and electron conductivity qualities.
Their poor volumetric and gravimetric energy densities are the fundamental drawback of the current flexible batteries. The stability of flexible electrodes is another challenge, particularly after repetitive stretching that could lead to fatigue and breaks in the electrodes.
Flexible LIBs can be extensively used in future electronics, despite the fact that numerous issues still need to be resolved. Future flexible LIBs will improve the comfort and design feasibility of wearable gadgets. Future flexible LIB applications and technical trends are depicted in Figure.
INTEGRATION
Future flexible LIBs need to be paired with other flexible devices, such solar cells, Nano generators, and different sensors, in addition to being utilized directly as the power source for flexible devices. The finished product might be produced more quickly and smoothly if the electronics and rechargeable batteries can be incorporated.
coupled LIB with dye-sensitized solar cells to create an integrated device that can handle energy harvesting and storing at the same time. Flexible LIBs must have great stretchability, a patterned shape, and a minimum thickness for these particular applications. As a result, the utilization of flexible LIBs heavily relies on both their multifunctionality and special flexibility. According to this viewpoint, the development of flexible LIBs depends on the inclusion of many functionalities into a single machine that can change with its surroundings.
MINIATURIZATION
The creation of flexible LIBs with minimal sizes is necessary for the construction of miniature electronic devices, including as microrobots and implantable medical equipment. The primary characteristic of miniatured flexible LIBs is their small area and thickness on flexible substrates. The manufacture of patterned thin-film electrodes using magnetron sputtering, spinning, and layer-by-layer assembly has advanced significantly in recent research on ultrathin planar batteries.
Thin-film electrodes typically have a thickness of a few micrometers or less. Graphene films can be micro patterned to create devices like an in-plane interdigital graphene micro-super capacitor on flexible substrates. A 3D micro-battery with a typical area of a few mm2 is another miniature LIB configuration that is particularly well suited for on-chip integration and tiny electronic devices. Using 3D printing technology, the 3D micro-battery was created by carefully interlacing stacks of small battery electrodes. These micro batteries, which can be as small as one millimeter square, are just as effective as regular batteries.
INTELLIGENCE
The idea of intelligent devices, which have some degree of interactive and autonomous functionality. Future flexible batteries should possess intelligence, which is a key component of both the Internet of Things and the Smart Planet. We can learn more about the operational states of smart and flexible batteries, and they can perform more new tasks like self-diagnosis, self-healing, self-control, and interactive connections with other smart devices, all of which can be controlled by software. An intelligent lithium-ion capacitor (LIC), for instance, has been created by Shan et al. with an extra lithium electrode serving as a voltage modulator.
This intelligent LIC performs self-regeneration and safety monitoring activities. By utilizing an internal Ni foil with one end attached to the negative terminal and the other extending beyond the cell to generate a third terminal, Wang et al. also developed a self-heating lithium-ion battery. Electrons start to pass through the nickel foil once the battery reaches a low temperature, which is detected by a temperature sensor connected to a switch. The battery's inside is rapidly warmed by this.
The user experience may be enhanced by these additional features, which give electrochemical energy-storage systems smart capabilities. They appear to be particularly attractive candidates for an intelligent power supply for smart, unmanned, and on-demand electronics, from nano-grids in devices to micro-grids for homes to industrial macro-grids, according to a review of a vast set of data.
TRANSPARENCY
Transparent flexible LIBs must be created in order to advance next-generation transparent electronics, such as roll-up touch-screen displays. Unfortunately, traditional LIB parts such packaging materials, separators, current collectors, and electrode materials are opaque. There are currently just two methods for creating transparent flexible LIBs. LiMn2O4 or Li4Ti5O12 thin films were deposited on transparent conducting oxides or polymer substrates as examples of how active materials can be made transparent by reducing their thickness until it is lower than their optical absorption length.
Transparent thin-film LIBs have relatively limited energy storage, nevertheless, because the light transmission of materials attenuates rapidly as thickness increases. As a result, the film of active material must be exceedingly thin. The usage of patterned grid-like battery electrodes on clear substrates, which only cover a small portion of the entire electrode, has recently been described as a novel design. This grid-like electrode exhibits some transparency without significantly reducing its ability to store energy.
The construction of the electrodes and batteries must be carefully planned in order to accomplish the aforementioned properties. Thus, a variety of fabrication techniques, including spinning, lithography, magnetron sputtering, and printing technologies, must be developed. Future flexible batteries will perform far better than those made today using the standard slurry-coating fabrication process, and they will depend more and more on nanotechnology.
In the end, fully developed flexible batteries may pave the way for a wide array of portable devices, including self-powered microrobots, smart watches, and integrated sensors. It's even possible that the mobile phones of the future may have a flexible, translucent body that will let sunlight charge the battery and illuminate the screen.
A relatively new area of study that is gaining popularity daily is flexible electronics. The need for wearable technology, biological sensors, and portable, flexible roll-up displays is increasing as a result of the advent of a new era of foldable power sources integrated with electronics. The functionality and convenience of bendable or even wearable devices is unmatched. In addition to having great electrochemical performance, they also significantly lessen the likelihood that the devices will malfunction or break under stress.
Flexible electronics have been theoretically possible for many years. In theory, anything long or thin can stretch to become flexible. Although flexible cables and wire are the best example, it wasn't until the space race that silicon wafers used for satellite solar cells were thinned to improve their power to weight ratio, allowing for some degree of warping. In the 1960s, the first flexible solar cells were made possible by this idea. Large steps toward flexibility and processability were made in the following decades with the development of conductive polymers, organic semiconductors, and amorphous silicon; as a result, these materials served as the foundation for electronic devices in applications requiring bending, rolling, folding, and stretching, among other properties that are not possible with conventional electronics.
New materials and fabrication methods that enable the direct creation of high-performance, scalable electronic devices on flexible substrates are currently of significant interest. As elastomeric substrates with potent molecular interactions are used, this interest has expanded to include not only flexibility but also features like stretchability and healability. Similarly, biocompatibility and biodegradability have been made possible by polymers that can be broken down into smaller constituent parts after use and have no negative effects on the body. With this new development, devices that can adapt to dynamic and complex surfaces, like those seen in biological systems and soft robots with a bioinspired design, are now possible.
CONCLUSION
New applications for these next-generation flexible electronics include flexible lighting and display technologies for consumer electronics, architecture, and textiles, wearables with sensors that track our habits and health, implantable electronics for better medical imaging and diagnostics, and enhancing the functionality of robots and unmanned aircraft with light-weight and conformable energy harvesting devices and sensor technology. Although conventional electronics are very capable of performing these tasks, flexible electronics are designed to increase the mechanical features to adhere to novel form factors through hybrid strategies, or as standalone solutions where the application does not call for high computation power. They are also meant to be extremely robust to deformation, low cost, thin, or disposable.
Depending on the context, flexibility might mean several things. Large area photovoltaics may be bent and rolled for simpler handling, while devices in electronic skin need to fold, twist, stretch, and deform in order to conform to unusual geometries while retaining device performance and durability. Although the field of flexible electronics has made some early strides and produced several significant discoveries, there are still many obstacles to overcome before it can be used in everyday life.
This represents a tremendous opportunity for scientific research and development to progress this field quickly and significantly.
Please feel free to reach out to one of our subject matter experts at info@effectualservices.com
to explore, how we can help you & make the world a better place to live in !!!
Effectual’s NANOTECHNOLOGY IN MEDICINE RESEARCH FRAMEWORK is a deep dive into this ecosystem and shall help you understand the intricacies of this nascent innovative domain with insights backed with credible data sources. Some ways we can help include, but not limited to - Performing any previous art or freedom to operate searches to help you better grasp the environment surrounding your invention or business endeavors. If certain methods of IP protection are more appropriate for your technological or business goals, we can help you strategize effectively to plan for future & in making continuous innovation a part of your working model